Cancer
In 2000, Douglas Hanahan and Robert Weinberg published the paper ‘The hallmarks of cancer’, conceptualising six core rules which orchestrate the multistep transformation of normal cells into malignant cells. More than 20 years later, in the third update ‘Hallmarks of cancer: new dimensions’, these six original hallmarks have expanded to 14. These include sustained proliferative signaling, evasion of growth suppressors, resistance to cell death, limitless replicative potential, angiogenesis, and genomic instability. Together, these hallmarks allow cancer cells to evade the normal controls that regulate cell growth and division, enabling them to continue to divide and proliferate, forming a tumor that can invade nearby tissues and spread to other parts of the body.
Fig. 1: Hallmarks of Cancer based on Hanahan and Weinberg
- Sustaining Prolifeerativae Signaling
- Evading Growth Suppressors
- Resisting Cell Death
- Limitless Replicative Potential
- Senescent Cells
- Deregulating Cellular Metabolsim
- Avoiding Immune Destruction
- Sustained Angiogenesis
- Activating Invasion & Metastasis
- Unlocking Phenotypic Plasticity
- Nonmutional Epigenetic Reprogramming
- Genome Instability & Mutation
- Polymorphic Microbiomes
- Tumor Promoting Inflammation
Hallmarks of Cancer:
Emerging Characteristics:
Sustaining Prolifeerativae Signaling
The most fundamental trait of cancer cells involves their ability to sustain chronic proliferation. This refers to the ability of cancer cells to activate signaling pathways that promote cell growth and division. Normally, these pathways are tightly regulated, but in cancer cells, they are constantly activated, allowing the cells to continue to divide and proliferate even in the absence of normal growth signals. This can be the result of mutations in genes that encode proteins involved in these signaling pathways, leading to the activation of downstream signaling molecules that promote cell growth.
Evading Growth Suppressors
A highly complementary hallmark capability for sustaining proliferative signaling in cancer cells is the ability to evade growth suppression. Growth suppressor genes are type of gene that normally function to inhibit cell growth and division. Several tumor suppressive protein-coding genes that operate in diverse ways to inhibit cellular growth and proliferation had been discovered. Prominent examples are the retinoblastoma protein (RB) or the human tumor suppressor p53. The transcription factor regulates the expression of genes involved in cell cycle control, induction of apoptosis (programmed cell death) or DNA repair after DNA damage. In cancer cells, these genes are often inactivated, either through mutations that disable their function or by mechanisms that prevent them from being expressed. This allows cancer cells to avoid the normal constraints on cell growth and division, enabling them to continue to proliferate unchecked.
Resisting Cell Death
In addition to evading growth suppressors, cancer cells also have a high degree of resistance to cell death. Normally, cells undergo programmed cell death, or apoptosis, when they become damaged or no longer needed. The cancer cells may alter the mechanisms that detect the damage or irregularities, preventing proper signaling and apoptosis activation. Cancer cells may also introduce defects in the downstream signaling itself, or the proteins involved in apoptosis, which would also prevent proper apoptosis. This can be the result of mutations in genes that regulate the apoptotic pathway, or it can be due to the activation of signaling pathways that promote cell survival.
Tumor Promoting Inflammation
Inflammation predisposes to the development of cancer and promotes all stages of tumorigenesis. Pathways evolved to mediate immunity to infection and promote tissue homeostasis, are usurped by tumors towards their benefit. Cancer cells as well as surrounding stromal and inflammatory cells form an inflammatory tumor microenvironment (TME). Additionally, inflammation induces the release of growth factors which support tumor growth.
There are several mechanisms of how the initial inflammatory responses may be induced. Examples are carcinogenic microbes, environmental pollutants and low-grade inflammation associated with obesity, as well epithelial barrier deterioration associated commensal microorganisms. The timing of cancer-associated inflammation is variable as well. Relevant causes and stimuli are autoimmunity or infection, malignant cells or can be triggered by anti-cancer therapy.
Inflammasome PathwayThe Tumor Microenvironment (TME)
The tumor microenvironment (TME) is the environment around a tumor, including the surrounding blood vessels, immune cells, fibroblasts, signaling molecules and the extracellular matrix (ECM). The tumor and the surrounding microenvironment are closely related and interact constantly. Tumors can influence the microenvironment by releasing extracellular signals, promoting tumor angiogenesis and inducing peripheral immune tolerance, while the immune cells in the microenvironment can affect the growth and evolution of cancerous cells.
Limitless Replicative Potential
The potential to replicate without limitation is another key aspect in tumor development which is also recognized as hallmark of cancer. In contrast to human body cells, cancer cells can overcome the ‘Hayflick Limit’ and divide indefinitely, without undergoing the normal process of cellular aging. Normally cells entering the state of senescence, or cell death stop replication. This is mainly due to the DNA at the end of chromosomes, known as telomeres. Telomeric DNA shortens with every cell division, until it becomes so short it activates senescence, so the cell stops dividing. Cancer cells bypass this barrier by manipulating the enzyme telomerase to increase the length of telomeres. Thus, they can divide indefinitely, without initiating senescence.
The Hayflick Limit
In mammalian cells the Hayflick Limit defines the amount of maximal cell divisions. After 60–70 doublings the cells reach a stage of senescence and multiplication is stopped. This limit can be overcome by disabling their Retinoblastoma (RB) and P53 tumor suppressor proteins, which allows them to continue doubling until they reach a crisis, with apoptosis or rare occuring emergence of an immortalized cell that can double without limit. Most tumor cells are immortalized.
Telomere decreases in size during each cell cycle. About 85% of cancers upregulate telomerase to extend their telomeres and the remaining 15% use alternative lengthening of telomeres.Senescent Cells
Cellular senescence is a typically irreversible form of proliferative arrest, likely evolved as a protective mechanism for maintaining tissue homeostasis, ostensibly as a complementary mechanism to programmed cell death that serves to inactivate and in due course remove diseased, dysfunctional, or otherwise unnecessary cells. Senescence can be induced in cells by a variety of conditions, including microenvironmental stresses such as nutrient deprivation and DNA damage, as well as damage to organelles and cellular infrastructure, and imbalances in cellular signaling networks.
Cellular senescence has long been considered a protective mechanism against tumors; whereby cancerous cells are induced to undergo senescence. Majority of triggers mentioned above are associated with malignancy, particularly DNA damage as a consequence of aberrant hyperproliferation, so-called oncogene- induced senescence due to hyperactivated signaling. However, new publications question this linear relationship. In certain contexts, senescent cells variously stimulate tumor development and malignant progression. The principal mechanism by which senescent cells promote tumor phenotypes is thought to be via senescence-associated secretory phenotypes (SASP).
Senescence-Associated Secretory Phenotype (SASP)
Multiple types of stimuli can provoke cellular senescence and a senescence-associated secretory phenotype (SASP). When irreversible cell-cycle arrest is triggered by severe DNA damage (i.e., dysfunctional telomeres or oncogenic stress), the SASP occurs in senescent cells. The acquisition of a SASP turns senescent fibroblasts into proinflammatory cells that have the ability to promote tumor progression. Senescent cells secrete interleukins, inflammatory cytokines, and growth factors that can affect surrounding cells. The most prominent cytokine of the SASP is interleukin-6 (IL-6), a pleiotropic proinflammatory cytokine. IL-6 has been shown to be associated with DNA damage– and oncogenic stress–induced senescence of mouse and human keratinocytes, melanocytes, monocytes, fibroblasts, and epithelial cells. Further, IL-6 secretion appears to be directly controlled by persistent DNA-damage signaling (ATM and CHK2), independent of the p53. Through IL-6 expression, senescent cells can directly affect neighboring cells.
Deregulating Cellular Metabolsim
For unhindered growth, tumors not only benefit from deregulated control of cell proliferation but also corresponding adjustments of energy metabolism to fuel cell growth and division. They have the capability to modify, or reprogram, cellular metabolism to support neoplastic proliferation most effectively. Under aerobic conditions, normal cells process glucose, first to pyruvate via glycolysis in the cytosol and thereafter to carbon dioxide in the mitochondria; under anaerobic conditions, glycolysis is favored, and relatively little pyruvate is dispatched to the oxygen-consuming mitochondria. Otto Warburg first observed an anomalous characteristic of cancer cell energy metabolism.
Warburg Effect
The ‘Warburg effect’ describes the reprogramming of glucose metabolism away from oxidative phosphorylation toward aerobic glycolysis, and it is one of the hallmarks of cancer cells. Several factors can be involved in this process, but in this review, the roles of non-coding RNAs (ncRNAs) are highlighted in several types of human cancer. ncRNAs, including microRNAs, long non-coding RNAs, and circular RNAs, can all affect metabolic enzymes and transcription factors to promote glycolysis and modulate glucose metabolism to enhance the progression of tumors. In particular, the 5′-AMP-activated protein kinase (AMPK) and the phosphatidylinositol 3-kinase (PI3K)/AKT/mammalian target of rapamycin (mTOR) pathways are associated with alterations in ncRNAs.
Avoiding Immune Destruction
Some cancer cells adapt mechanisms to evade immune surveillance and attack by the host's immune system. One way cells do this is by hijacking normal mechanisms of immune checkpoint control. Immune checkpoints refer to the built-in control mechanisms of the immune system that maintain self-tolerance and help to avoid collateral damage during a physiological immune response. Tumor-specific T cells must discriminate between destruction of the tumor cell and survival of the target cell. Important for discrimination are proteins on both the T-cell and the target cell. Tumor cells express molecules to induce apoptosis or to inhibit T lymphocytes: PD-L1 on the surface of tumor cells leads to suppression of T lymphocytes. FasL on the other hand may induce apoptosis of T lymphocytes. Some cancer cells also try to gain resistance against possible cytotoxic CD8+ T cell which are a fundamental element of anti-tumor immunity. They lower their MHC I expression and avoid being detected by the cytotoxic T cells. Disruption of the apoptotic signal pathway molecules also leads to successful immune evasion by the tumor. Caspase 8, Bcl-2 or IAP are key targets, amongst others.
Sustained Angiogenesis
An expanding tumor has an increased need for nutrients to sustain his growth and spread. The tumor requires new blood vessels to deliver adequate oxygen to the cancer cells. To do this, the cancer cells acquire the ability to undergo angiogenesis, the process of forming new blood vessels. Cancer cells orchestrates the production of new vasculature by releasing signaling molecules that activate the 'angiogenic switch'. By exploiting the switch, non-cancerous cells that are present in the tumor are stimulated to form blood vessels.
Tissue Inhibitors of Metalloproteinases (TIMPs)
Four members of the TIMP family are able to inhibit matrix metalloproteinases (MMPs) by forming tight-binding, noncovalent associations with the active site of the MMPs. MMPs facilitate tumor cell invasion and metastasis by at least three distinct mechanisms. First, proteinase action removes physical barriers to invasion through degradation of ECM macromolecules such as collagens, laminins, and proteoglycans. Second, MMPs have the ability to modulate cell adhesion, crucial for cell movement through the ECM. The ratio of MMP-2 to TIMP-2 significantly impacts variation in the adhesive phenotype of tumor cells. Finally, MMPs may act on ECM components or other proteins to reveal biologic activities. For example, the angiogenesis inhibitor angiostatin may be produced from plasminogen by MMP action and laminin-5 is specifically degraded by MMP-2 to produce a soluble chemotactic fragment. Thus MMPs play multiple key roles in facilitating the metastasis of tumor cells.
Activating Invasion & Metastasis
The ability to invade neighboring tissues is what dictates whether the tumor is benign or malignant, and it renders cancer a mortal threat. Metastasis enables their dissemination around the body complicates treatment by a big margin. The cancer cells must undergo a multitude of changes in order for them to acquire the ability to metastasize. The metastatic cascade represents a multi-step process which includes local tumor cell invasion, invasion of blood vessels followed by the exit of carcinoma cells from the circulation and colonization in the new tissue. At the earliest stage of successful cancer cell dissemination, the primary cancer adapts the secondary site of tumor colonization involving the tumor-stroma crosstalk.
Cytokeratins in Tumor DetectionEpithelial- Mesenchymal Transition
The ‘Epithelial- Mesenchymal Transition’ (EMT), a process involved in various steps of embryonic morphogenesis and wound healing, is being used by carcinoma cells to acquire multiple attributes that enable invasion and metastasis. This multifaceted EMT program can be activated transiently or stably, and to differing degrees, by carcinoma cells during the course of invasion and metastasis. The transition is an example for the hallmark enabling characteristic ‘phenotypic plasticity’. Transcriptional factors, including Snail, Slug, Twist, and Zeb1/2, orchestrate the EMT and related migratory processes during embryogenesis. They are also expressed in various combinations in a number of malignant tumor types and are involved in carcinoma formation and for programming invasion.
Unlocking Phenotypic Plasticity
Relative to the huge amount of development and differentiation that occurs during organogenesis, mammalian cells are typically restricted in the extent to which they can differentiate. This restriction allows cells to remain organized and functional within their respective tissue. In cancer, however, cells undergo molecular and phenotypic changes that allow them to adopt different identities along a phenotypic spectrum referred to as cellular plasticity. This includes dedifferentiation from mature to progenitor states, blocked differentiation from progenitor cell states, and transdifferentiation into different cell lineages. The EMT and mesenchymal-to-epithelial (MET) transitions are well-characterized examples of developmental regulatory programs that resemble transdifferentiation. Changes in cellular phenotype are important to cancer progression because these changes can facilitate tumor initiation and metastasis, immune invasion, chemoresistance and many other aspects of tumor progression.
Genome Instability & Mutation
Genomic instability and thus mutability endow cancer cells with genetic alterations that drive tumor progression. Certain mutant genotypes give subclones of cells a selective advantage and enable them to spread and eventually dominate in a local tissue environment. The genome repair machinery in mammalians detects and resolves defects in the DNA and ensures that rates of spontaneous mutation are usually very low during each cell generation. In order to increase the number of mutant genes needed to orchestrate tumorigenesis, cancer cells try to increase the rates of mutation. Increased sensitivity to mutagenic agents and breakdown of the genomic maintenance machinery in several components are main drivers to increase the mutability. Defects in the DNA-maintenance machinery—often referred to as the ‘caretakers’of the genome—positively correlate with human cancer development. The so called ‘‘guardian of the genome’’, p53, plays the central role in the machinery, so does telomerase.
Nonmutional Epigenetic Reprogramming
In the big picture of hallmarks of cancer, ‘nonmutational epigenetic reprogramming’ is viewed as an emerging characteristic. It is an additional, apparently independent mode of genome reprogramming that involves purely epigenetically regulated changes in gene expression. Changes in gene and histone modification, in chromatin structure, and in the triggering of gene expression switches that are stably maintained over time by positive and negative feedback loops are summarized as epigenetic alterations. They regulate gene expression in developmental and adult cells. Growing evidence supports the proposition that analogous epigenetic alterations can contribute to the acquisition of hallmark capabilities during tumor development and malignant progression.
Nonmutational epigenetic reprogramming is key for enabling the hallmark capability of phenotypic plasticity mentioned above. The epigenetic reprogramming results in dynamic transcriptomic heterogeneity, a feature of cancer cells populating malignant TMEs. ZEB1, master regulator of the EMT, is a good example for nonmutational epigenetic reprogramming. It induces expression of a histone methyltransferase, SETD1B, that in turn sustains ZEB1 expression in a positive feedback loop that maintains the (invasive) EMT regulatory state. Similarly, upregulation of the transcriptions factor SNAIL1 due to chromatin landscape alterations induces EMT build up. The chromatin modifiers responsible for the alterions are demonstrably necessary for the maintenance of the phenotypic state.
Polymorphic Microbiomes
As studies mount, there is more and more evidence that the ecosystems created by resident bacteria and fungi—the microbiomes—have profound effects on health and disease. The gut microbiome has been the pioneer of this new frontier, multiple tissues and organs have associated microbiomes, which have distinctive characteristics in regard to population dynamics and diversity of microbial species and subspecies. In relation to cancer, there is increasingly strong evidence that polymorphic variability in the microbiome between individuals in a population can influence the cancer phenotype. Microorganisms, principally but not exclusively bacteria, may be directly carcinogenic, impact host immune responses to promote malignancy, and may be key effectors in determining the efficacy of anticancer therapy. Manipulation of the microbiome is showing promise as an opportunity to influence cancer outcomes.
Butyrate-producing bacteria are an example for specific bacterial species promoting tumorigenesis. Studies indicated that mouse models as well as patients with colon carcinogenesis populated with butyrate-producing bacteria developed more tumors than mice lacking such bacteria. The production of the metabolite butyrate has complex physiologic effects, including the induction of senescent epithelial and fibroblastic cells.
References
- The hallmarks of cancer." in: Cell, Vol. 100, Issue 1, pp. 57-70, (2000) (PubMed). : "
- Hallmarks of cancer: the next generation." in: Cell, Vol. 144, Issue 5, pp. 646-74, (2011) (PubMed). : "
- Hallmarks of Cancer: New Dimensions." in: Cancer discovery, Vol. 12, Issue 1, pp. 31-46, (2022) (PubMed). : "
- DNA methylation of tumor suppressor protein-coding and non-coding genes in multiple myeloma." in: Epigenomics, Vol. 7, Issue 6, pp. 985-1001, (2016) (PubMed). : "
- Cell cycle, oncogenic and tumor suppressor pathways regulate numerous long and macro non-protein-coding RNAs." in: Genome biology, Vol. 15, Issue 3, pp. R48, (2015) (PubMed). : "
- Alternative lengthening of telomeres: from molecular mechanisms to therapeutic outlooks." in: Cell & bioscience, Vol. 10, pp. 30, (2020) (PubMed). : "
- Inducing Angiogenesis, a Key Step in Cancer Vascularization, and Treatment Approaches." in: Cancers, Vol. 12, Issue 5, (2020) (PubMed). : "
- Matrix metalloproteinases and metastasis." in: Cancer chemotherapy and pharmacology, Vol. 43 Suppl, pp. S42-51, (1999) (PubMed). : "
- Polymorphic microbes: a new emerging hallmark of cancer." in: Trends in microbiology, Vol. 30, Issue 12, pp. 1131-1134, (2022) (PubMed). : "
- Initial steps of metastasis: cell invasion and endothelial transmigration." in: Mutation research, Vol. 728, Issue 1-2, pp. 23-34, (2011) (PubMed). : "
- Emerging role of tumor cell plasticity in modifying therapeutic response." in: Signal transduction and targeted therapy, Vol. 5, Issue 1, pp. 228, (2021) (PubMed). : "
- The gut microbiome switches mutant p53 from tumour-suppressive to oncogenic." in: Nature, Vol. 586, Issue 7827, pp. 133-138, (2021) (PubMed). : "
- Microbes and Cancer." in: Annual review of immunology, Vol. 35, pp. 199-228, (2018) (PubMed). : "
- The Warburg Effect: How Does it Benefit Cancer Cells?" in: Trends in biochemical sciences, Vol. 41, Issue 3, pp. 211-218, (2016) (PubMed). : "
- Regulation of Glycolysis by Non-coding RNAs in Cancer: Switching on the Warburg Effect." in: Molecular therapy oncolytics, Vol. 19, pp. 218-239, (2020) (PubMed). : "
- Inflammation and Cancer: Triggers, Mechanisms, and Consequences." in: Immunity, Vol. 51, Issue 1, pp. 27-41, (2019) (PubMed). : "
- Unmasking senescence: context-dependent effects of SASP in cancer." in: Nature reviews. Cancer, Vol. 19, Issue 8, pp. 439-453, (2019) (PubMed). : "
- On the evolution of cellular senescence." in: Aging cell, Vol. 19, Issue 12, pp. e13270, (2021) (PubMed). : "
- Genomic instability--an evolving hallmark of cancer." in: Nature reviews. Molecular cell biology, Vol. 11, Issue 3, pp. 220-8, (2010) (PubMed). : "
- Epithelial-to-Mesenchymal Transition: Epigenetic Reprogramming Driving Cellular Plasticity." in: Trends in genetics : TIG, Vol. 33, Issue 12, pp. 943-959, (2018) (PubMed). : "
- The tumor microenvironment." in: Current biology : CB, Vol. 30, Issue 16, pp. R921-R925, (2021) (PubMed). : "
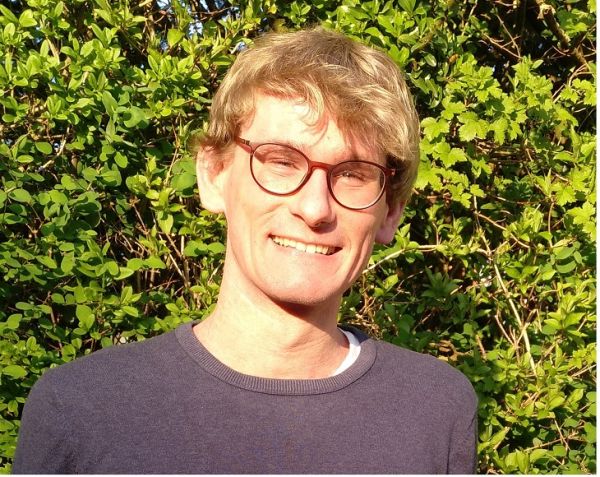
Creative mind of antibodies-online with a keen eye for details. Proficient in the field of life-science with a passion for plant biotechnology and clinical study design. Responsible for illustrated and written content at antibodies-online as well as supervision of the antibodies-online scholarship program.
Go to author page